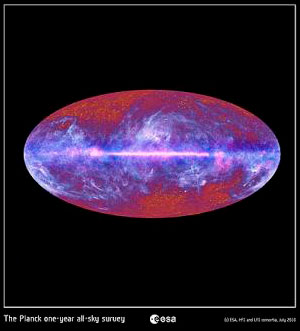
The Observational Cosmology and Instrumentation group at IFCA focuses on the study of several topics related to the origin and evolution of the Universe. Our research accounts for the analysis and interpretation of astronomical data, as well as for the design and development of instrumental devices to perform the measurement of these data.
RESEARCH TOPICS
Our major research topics are:
GAUSSIANITY AND ISOTROPY OF THE COSMIC MICROWAVE BACKGROUND
Current observations tell us that the content, evolution and dynamics of the Universe seem to be well described by a
concordance model in which baryonic particles account for only 15% of the matter in the Universe, whereas the remaining 85% appears in the form of non-relativistic, non-baryonic and weakly interactive particles (the
Cold Dark Matter). Within the concordance model, matter only represents around 25% of the energy content in the Universe: the dominant 75% is the so-called
Dark Energy, which is responsible for the current accelerating expansion of the Universe.
Among the observations that had led to this consensus picture, the
Cosmic Microwave Background (CMB) data (a relic electromagnetic radiation coming from an early stage of the Universe evolution) have played a central role. One of the fundamental milestones achieved by the analysis of the CMB observations is the overall agreement of the CMB statistical properties with the predictions made by the
Cosmic inflation, within the framework of the
Cosmological Principle of
homogeneity and
isotropy of the Universe.
However, the picture is not yet fixed. One of the most interesting research topics in the cosmology field is, indeed, to check whether the concordance model is sufficient or not, to explain the properties of the CMB temperature fluctuations. In particular, it has been argued that
alternative scenarios (like
non-standard inflation,
topological defects or
anisotropic models) might still play a secondary role in the picture of the Universe evolution.
It is precisely in this context where the study of the Gaussianity and isotropy of the CMB signal is crucial. Most of the alternative/complementary scenarios to the standard model predict different levels of Gaussianity and/or isotropy departure. The development and application of sophisticated
image
processingtechniques and
statistical approaches to CMB data is one of our main research topics.
COMPONENT SEPARATION OF THE MICROWAVE SKY
As it was said above, the
CMB is a
very valuable observation to
disentangle some of the cosmological
properties of the Universe. However, to extract this information from the microwave observations it is not an easy task.
The global pipeline that goes from the observations to the final products, like cosmological parameters and the constraints on the compelling for the formation and evolution of the Universe, is a real challenge.
One of the most difficult steps of this chain is the issue of
separating the
emission due to the CMB from other astrophysical sources that also emitted at microwave frequencies. This activity is the so-called,
component separation process. It is also remarkable that this process is not only interesting just because the cleaning of the CMB, but also because provides very
useful information about some other
physical phenomena that are poorly studied.
The complexity of the source separation problem is very high, and several approaches have been proposed, depending on the kind of solution that one is looking for. To better understand the problem, it is worth to mention that the characteristics and the statistical properties of the different components that form the microwave sky are very heterogeneous. Beside the cosmological emission due to the CMB photons, there are several microwave emissions due to different physical phenomena that take place in our Galaxy and someothers produced by other galaxies and by clusters of galaxies. Among the former, the most important ones are the synchrotron radiation emitted by the charged particles interacting with the magnetic field of the Galaxy, the free-free emission produced by the deceleration of an electron that interacts with an ion, and the thermal and dipolar emission produced by the dust grains of the interstellar medium. These diffuse emissions appear concentrate on the galactic plane, and they present a large scale structure. On the other hand, the contaminant emission due to dusty and radio galaxies and to the Sunyaev-Zeldovich effect (SZ) produced by the CMB photon interaction with the hot and dense electron gas in clusters of galaxies, appear, however, as point-like objects, homogeneously distributed in the sky.
As it was mentioned above, the variety of emissions (CMB, diffuse galactic ones and point-like objects) has addressed to a picture where several tools are used, depending whether one is interested on recovering the CMB, the galactic emissions, the extragalactic point sources and the cluster of galaxies or everything at the same time.
Our group have large experience on the development and application of devoted tools (based on wavelets –like the
Mexican Hat Wavelet Family– and optimal filters) to detect the point-like objects. In addition, we have also developed a code (SEVEM) focused on the recovery of the CMB emission.
LARGE SCALE STRUCTURE AND SECONDARY ANISOTROPIES
We work in several aspects related to the analysis of the large scale structure (LSS) of the Universe.
One the one hand, we have performed
N-body simulations of large volumes (300 Mpc/h) with and without gas particles in order to estimate the
Rees-Sciama effect. The derivative of the potential is computed in these boxes and then projected along the line of sight with the observer at the centre of the box at redshift 0. These simulations can be used in studies looking at techniques to detect the ISW and/or Rees-Sciama effects. We have also computed the gravitational lensing effect. We also work on simulations of the high redshift Universe with a focus on the reionization period and the opportunities to study this epoch with future instruments.In addition to the simulation work, we also investigated on optimal
cross-correlation tools to extract the common signal that is present on the CMB maps and on the LSS surveys (due to the gravitational potential evolution). In particular, we were
piooners by introducing wavelets in this field. The scientific outcomes produced by these analyses is twofold. First, it probes the common physical origin between the CMB photons emitted at redshift 30000 and the matter distribution up to redshift around 2. Second, it helps to put constraints to the
cosmological parameters that define the
Dark Energy properties.
GRAVITATIONAL LENSING
We also research on the study of galaxy clusters through the
gravitational lensing (GL) effect. Galaxy clusters contain large amounts of dark matter and GL can map the mass of the cluster. As a consequence through GL it is possible to weight the amount of dark matter once the mass from the galaxies and the gas is taken into account. The group has made
pioneering work in the area of microlensing near cluster caustics, a technique that is being used to constrain the amount of dark matter in compact form, with masses ranging from planetary masses to dwarf halos. This technique will be used extensively with the future JWST telescope where proposals to observe the most distant stars and map the smallest susbstructures in clusters are already in place.
SIGNAL PROCESSING AND STATISTICS
A common task that is of crucial importance to all the previous research projects is the statistical analysis of the different astrophysical signals in order to obtain useful information about physical phenomena.
Statistical signal processing is the branch of mathematics that treats signals as stochastic processes, dealing with their statistical properties as a means to derive rules from phenomena that apparently evolve in time in an unpredictable manner. Examples of typical statistical signal processing tasks that have already been mentioned here are
image processing,
model selection,
component separation and
source detection.
In this era of technologically ambitious experiments, we need to process huge loads of data in a fast and robust way. Besides, astrophysical data is usually very complex (multiwavelenght observations, polarization data, non-stationary and non-Gaussian physical processes, complicated systematics of the instrument, etc). For this reason it is important to develop appropriate data processing techniques and to implement them in the
supercomputing
facilities we have access to in the IFCA. Some of the hot topics we are working with are
Bayesian inference,
time-frequency analysis,
linear and
non-linear filtering,
image fusion,
wavelets,
sparse representations,
Markov random fields,
non-linear sampling,denoising and
inverse problems.
Our main goal is to use existing signal processing techniques and to develop new ones to be applied to the study of the Cosmic Microwave Background and the sub-mm Universe, but we are not restricted to thas field. Many of the applications we are working with can be useful in other knowledge areas such as
telecommunications,
geophysics,
bioinformatics and
biomedical applications.
INSTRUMENTATION TOPICS
Our research on instrumentation for microwave experiments follows three different lines:
MICROWAVE (MW) RADIOMETERS
We have implemented efficient modelling methods with application to the QUIJOTE experiment 30 and 40 GHz radiometers. Efficient models have been obtained from the different subsystems of the radiometer (low noise amplifiers, band pass filters, hybrids, cables and detectors). These models allow realistic simulations of the overall receiver both in time and frequency domain, using Gaussian noise as excitation signals. Simulation studies under nominal conditions provide results very similar to the actual ones, helping in the design stage and also to the analysis of eventual issues during instrument operations. Taking into account that QUIJOTE instruments have been designed to measure CMB polarization, time domain simulations are very helpful to verify accurately the polarimeters behaviour when observing the sky and extracting Stockes parameters (I, Q and U). These simulations also give very important information about the non-linear behaviour of some radiometer subsystems, due to its dependence with the observed signal characteristics.
NEAR-INFRA-RED (NIR) ELECTRO-OPTICAL (EO) TECHNOLOGY FOR MW INSTRUMENTATION
In the frame of the EPI-Consolider project, a viability study was performed to analyse the optimal technology for the implementation of a correlator for a large-format MW interferometer with hundreds or even thousands of receivers. During the study digital, analogical and NIR optical technologies were analysed. Taking into account the complexity provided by the correlation of hundreds of MW signals, NIR-EO technology was selected as the most promising to achieve a correlator for a large-format MW interferometer operating in the QUIJOTE experiment frequency bands (10-50 GHz).
The basic idea is to modulate L band (1550 nm) laser signals with the CMB microwaves using Mach-Zehnder optical modulators (MZM), to route and correlate the signals by means of an optical system based on fibres, lenses and near-infrared (NIR) cameras. Our goal is to reuse the QUIJOTE experiment receivers and the optical correlator to validate the proposed idea.
The main bottleneck of the optical correlator concept is the use of wideband commercial MZM due to the high cost of the LiNbO3 technology. We are leading a “Plan Estatal Explora-Tecnología” project to get an integrated version of a frequency up-conversion stage based on MZMs implemented in a technology allowing integration as InP to assure the viability of the proposed instrumentation.
The same technology can be also applied to implement the receivers of MW imaging instruments, designing and implementing the corresponding MW circuits as Photonic Integrated Circuits (PIC). This will serve to overcome systematic errors provided by the wide relative bandwidths of the receivers (the same bandwidths are very narrow when are translated to the NIR wavelengths) and also to reduce the cost by taking advantage of the integration capabilities of the optical technology. In this sense, a new “Plan Estatal I+D” project has been approved to study PIC technology capabilities.
CALIBRATION OF CMB EXPERIMENTS
In the frame of present and future high sensitivity CMB experiments, the calibration of the instrumentation with high accuracy is fundamental to reduce systematic errors to the level required to be able to exploit that sensitivity. The specific calibration technique depends on the particular instrument topology and detection technology. Our group participates in the calibration and commissioning phase of the QUIJOTE experiment instruments, that are polarimeters operating in the 10-50 GHz frequency range and based in ultra-wide band heterodyne MW receivers.
For QUIJOTE some galaxies, natural satellites and planets can be used as calibration sources during commissioning and operational phases, but for other experiments presenting much more sensitivity levels, as the proposed space missions -CORE (ESA), LiteBIRD (JAXA), PICO (NASA)- but also ground-based (S4) and balloon-borne (Spider) experiments, the use of very stable and well known artificial sources is fundamental to reduce systematic errors to the required levels.
In that sense, we have proposed an H2020 project (CMBCalSys) with the objective of developing a novel calibration system for existing and future CMB polarization experiments.
For space missions, the specific objective of the project is the detailed design of an artificial calibrator operating on a small ancillary satellite, either a CubeSat or a nano-satellite, flying in formation with the main satellite. For ground-based and sub-orbital experiments we aim to design a calibrator flying on a Low Earth Orbit (LEO) satellite, as well as on an aerial drone. A drone will be particularly effective for ground-based telescopes whose far field is between a few tens and hundreds of meters. Our artificial calibrators will operate within view of the telescopes, providing a calibration source in the far field of the fully operative polarimeters. As a result, the observations will benefit from our absolute calibration on the polarization angle and will allow the measurement of the detector beam response, meeting the accuracy requirements for the next generation of CMB experiments. This would be the first study of an experimental calibration method working during a satellite mission operations phase.
For all those experiments, the detection technology is based on TES (Transition Edge Sensors) or KID (Kinetic Inductance Detectors) technology. As KID technology is being developed rapidly in Europe and particularly with the collaboration of the Centro de Astro-Biología (CAB), in Madrid, in the frame of our new funded “Plan Estatal I+D” project we will develop an specific calibration set-up to be applied in the laboratory to high sensitivity cameras based on KID technology.
Follow this link to check the group publications.
Group Projects
Group Members
Group Publications